Achieving reliable results in tensile testing of plastics
Automotive and medical applications increasingly call for tighter tolerances, requiring suppliers to perform their own testing. Tensile testing is commonly used, and represents an integral input to quality control.
September 14, 2017
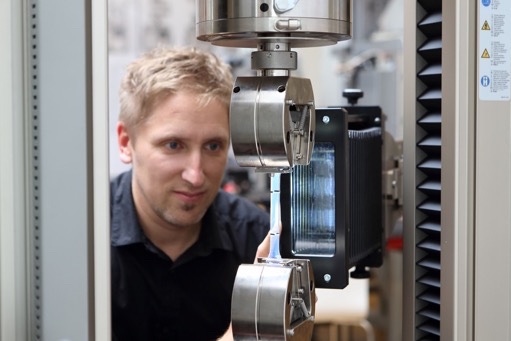
Plastics compounders routinely perform mechanical tests as part of the product development cycle. Producers often turn to universal testing machines (UTMs) to pull, stretch, bend or compress test specimens to determine the suitability of a material for specific process and end-use applications. They also use UTMs for production quality control and as a final checkout to release customer shipments. Applications for compounds have grown increasingly complex, particularly in the medical and automotive industries. Such changes have inspired an increased emphasis on accuracy in measurement.
Today, plastics molders and extruders also use UTMs to evaluate the quality of incoming materials as well as the performance of parts at the end of production runs. Supply chains have evolved over the past 10 years, with processors playing a greater role in upfront product and process development. Close coordination with OEMs has enabled the production of increasingly complex parts and components, as well as the introduction of advanced materials with unique engineering properties. Stricter specifications on performance have led processors to increase focus on the validation of key properties. This has created demand in the market for capabilities that reduce errors in testing.
“Applications in the automotive and medical device industries call for tighter tolerances. OEMs in these sectors require suppliers to perform their own testing and to include spec sheets in lot shipments. Engineering properties must be thoroughly validated, and tensile testing represents one of the most common tests customers perform,” says Boris Plach, Product Manager for Zwick/Roell (Ulm, Germany).
The Midwest’s largest advanced manufacturing event is coming to Minneapolis in November. Six co-located events, including PLASTEC Minneapolis and Medical Design & Manufacturing (MD&M) Minneapolis, will welcome more than 600 suppliers and thousands of attendees to the Minneapolis Convention Center on Nov. 8 and 9, 2017. Go to the event website for more information and to register to attend. |
The test method for determining the tensile properties of plastics is outlined in ISO 527-1/-2:2012. In the standardized tensile test, results are based on a defined pull-off speed on the specimen. The loads on a component or structure in actual service, however, may lie within a very wide range of the deformation rate. Due to the visco-elastic properties of polymers, mechanical properties different from those measured on a standardized test specimen normally result under altered strain rates. For this reason, the characteristic values determined in a tensile test are only of limited suitability for component design, but represent a very reliable basis for material comparisons. The tensile test, therefore, represents an integral input to quality control.
While tensile testing is common among compounders and processors alike, achieving reliable test results can pose a challenge. Multiple factors influence the reliability of test data; gaining an understanding of the areas that directly impact this is integral to quality management.
There are four components to the achievement of reliable test results: Accuracy, repeatability, reproducibility and traceability.
Accuracy in measurement
Measurements that are true and precise are accurate. Trueness describes the closeness of agreement between a reference value and the average of individual test results. Precision is the closeness of agreement between individual test results obtained under stipulated conditions; however, this still does not mean that the values obtained by measurement are also true. They can be precisely untrue, for example, due to systematic error.
Testing machines measure two fundamental values: Force and extension. As part of periodic calibration when compared to a measuring instrument based on international standards, evidence has shown that these measured values achieve a level of accuracy defined in the test standard across defined measuring ranges.
Force measurement to ISO 7500-1 and ASTM E4
Most test standards require a measurement accuracy of 1% for the measured value. This requirement is categorized as Class 1 in the ISO environment. Almost all modern testing machines today achieve Class 1 accuracy, or even Class 0.5 with tolerances that are halved. Various Zwick testing machines achieve Class 1 at as little as 1/1000 of their measurement range. This means measuring the modulus values and tensile stress of many materials is possible with the same test arrangement and without the need to reconfigure the arrangement.
Extension measurement to ISO 9513 and ASTM E83
Along with a defined relative error (expressed in percentile), the class specifications for measuring extension also include a specification for an absolute error, which occurs when measuring smaller extensions (Fig. 2). ISO and ASTM differ here significantly. While ISO tolerances refer to the extension, ASTM makes direct reference to strain. Furthermore, the requirements for smaller strains are defined more narrowly in ISO than in the corresponding ASTM class. Depending on the gage length used, this sometimes results in significant differences by definition, particularly when measuring small extensions.
Fig. 1: Accuracy requirements for the strain range of the tensile modulus, as specified by ISO Class 1. |
As seen in Figure 1, the accuracy requirements for the strain range of the tensile modulus in ISO Class 1 are ±3 µm. This means that a deviation of up to 6 µm may exist between measurements at the beginning and end of the modulus range. This would result in a correspondingly large measurement error. To address this, an additional requirement for measuring the tensile modulus was added to ISO 527-1. This additional requirement states that the measurement path between the beginning and end of modulus determination must be measured with an accuracy of 1% (Fig. 2). Zwick testing machines operate in conformance to ISO 527-1.
Fig. 2: Specification of ISO 527-1 indicating that the measurement path between the beginning and end of modulus determination must be measured with an accuracy of 1%. |
Tensile modulus measurements in accordance with ISO 527 require the use of an extensometer. High-accuracy contact and non-contact extensometers, such as the makroXtens and videoXtens, respectively, sense deformations well below 1 µm.
Clip-on and sensor arm extensometers are in direct mechanical contact with the specimen via knife edges that are perpendicular to the gage length. Sensor arm extensometers offer the advantages of automatic operation and a large measurement range with high measurement accuracy. Precision designs with a very smooth and balanced mechanical operation apply minimum loading to the specimen (as little as the measurement marks used for noncontact extensometers). Because the sensor arms are in contact with both sides of the specimen, superimposed bending strains are largely compensated. Sensor arm extensometers offer extremely high accuracy, excellent repeatability and ease of use due to fully automatic operation, which includes the setting of variable gage lengths.
A primary advantage of noncontact video extensometers is that they may be used up to the material breaking point without damage. Another advantage is the capability to more accurately determine strain and use strain as a control loop mechanism for test samples undergoing large deformations.
Repeatability—a pivotal factor
The concept of repeatability means demonstrating precision under repeat conditions, specifically:
the same measurement procedure;
the same operator;
the same measuring equipment;
the same location; and
the same test conditions.
Maintaining high levels of repeatability poses challenges for busy test labs managing throughput requirements. Changeovers from one to test to another have historically presented opportunities for inadvertent errors to emerge.
“The System Configuration Builder in our testXpert III testing software saves all relevant machine settings and assigns them to a user-defined test environment. This guarantees an identical test configuration every time, enabling optimum test result repeatability,” explains Plach.
The role of reproducibility in comparison studies
The overriding goal of testing molding materials is to achieve a high degree of reproducibility. When tests are reproducible, they support material comparison studies. Limiting the number of specimen types enables tests to be performed with high levels of reproducibility.
Specimens are usually produced by injection molding. In ISO 527-2, specimens are designated as Type 1A. In ISO 3167, these are designated as Specimen Type A and are additionally restricted to a specified thickness of 4 mm. These specimens are also designated as Type A1 in ISO 20753.
Injection molded specimens display decreasing orientation as the distance from the feed point increases, leading to non-constant mechanical property curves along the length of the specimen, and, therefore, frequently resulting in specimen break on the side away from the gate. The preferred gage length for the specimen is 75 mm, or alternatively 50 mm. Alternately, Type 1B specimens are permitted; these are designated as Type B in ISO 3167 and as Type A2 in ISO 20753. They are generally machined from pressed or injection molded sheets. The orientations of the polymer normally differ significantly from those in injection molded specimens. Comparability of results obtained using different specimen shapes is not guaranteed. A gage length of 50 mm is specified for Type 1B specimens due to the larger radius resulting in a shorter parallel area.
Improper determination of test specimen dimensions can result in a relatively high number of stress value errors. When a specimen is subjected to a tensile load, the measurement error is reflected linearly by the stress result. When a specimen is subjected to a flexure load, the specimen thickness measurement error has a quadratic effect. In addition to the reading accuracy of the measuring equipment, the size and form of the contact element and the applied surface pressing during measurement also play an important role. Furthermore, the cross-section of the specimen often differs from an ideal rectangular form. This could be angular errors resulting from mechanical processing or sink marks and minor draft angles in injection molded specimens.
Many test standards refer to ISO 16012 or ASTM D5947 to define the requirements and methods of dimension measurement. Sometimes, individual test standards contain additional specifications. For example, a caliper is normally used to measure the overall length of rigid plastics larger than 10 mm. Since surface pressing during measurement cannot be checked, measurement accuracy is rather low even if the resolution of the caliper is high. The thickness and width of the specimen is normally determined by a micrometer screw with a ratchet. The contact surface is flat and circular with a diameter of 6.35 mm. The ratchet limits the measurement force to 5 to 15 N. In automated systems, the thickness and width is determined by a cross-section measuring device. This device holds the specimen during measurement and determines the dimensions with four digital measuring transducers, a defined measurement force and sensor feet. For soft plastics and films, it is imperative that the measurement force is strictly observed. To ensure this, digital thickness measuring instruments with dead weight supports should be used.
Fig. 3: TestXpert testing software from Zwick lends robust support for traceability through electronic logging and documentation of all processes and events. |
Traceability and support for recordkeeping
Increased levels of stringency on the performance of plastics and the need to retain full documentation of measured results in the event of an external audit have inspired a greater emphasis on traceability. Inputs gathered to support traceability include information on by whom, how, when and where the test result was determined. Traceability of data is achieved through electronic logging and documentation of all processes and events on Zwick testing machines. This is accompanied by an accurate time-stamp, which is assigned to the operator (Fig. 3).
“Intelligent user management in our testXpert testing software controls all access rights and safeguards data against manipulation,” explains Plach.
The achievement of reliable results is the cornerstone of all testing programs. Whether a program is focused on delivering vital inputs for batch-to-batch comparisons or assessing adherence to manufacturing processes and protocols, test machines from Zwick are engineered to meet the demands of compounders and processors alike.
Margaret Johns is President of Bluewater Consulting, a specialized business consultancy serving enterprises in the plastics, composites, automotive, aerospace, industrial machinery and test and measurement markets. Johns assists companies across the plastics value chain with growth initiatives. From market analysis and positioning to channel selection and management, she leads engagements that focus on rapid delivery of value. Prior to co-founding Bluewater Consulting, Johns was Global Sales and Marketing Manager for the nano-mechanical testing business of Agilent Technologies. She can be reached via e-mail at [email protected].
About the Author(s)
You May Also Like